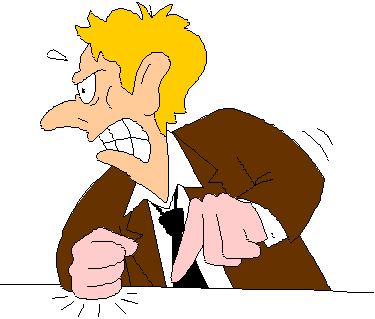
Control of Breathing
- Control of breathing is effected by intrinsic rhythmicity modified by neural and chemical input.
- Final common pathway: phrenic nerves, nerves to intercostals, etc., Pulmonary ventilation is therefore determined by:
- The interval between successive groups of nerve discharges to the muscles of inspiration (and expiration, if active). This determines the rate of respiration.
- The frequency of the nerve impulse transmitted by the individual nerve fiber to its motor unit.
- The duration of nerve fiber activity.
- The number of motor units activated during each inspiration or expiration.
- Respiratory center - anatomically diffuse, functionally integrated.
- Rhythmic pattern of breathing
- Medullary Center
- Inspiratory and expiratory neurons located in reticular formation of medulla beneath the lower part of the fourth ventricle.
- Spontaneous activity - can section brain above the respiratory center and still get rhythmic inspiration and expiration
- These do not appear to be discrete areas of expiratory and inspiratory neurons (as often seen in texts) but are anatomically intermingled. There appear to be two dense bilateral aggregations of respiratory neurons.
- Dorsal respiratory groups (DRG). Located in the ventrolateral portion on the nucleus of the tractus solitarius. Primarily inspiratory cells.
- These inspiratory neurons project primarily to the contralateral spinal cord and drive the diaphragm via the phrenic nerves. DRG units also project to the VRG.
- The nucleus of the tractus solitarius is a primary projection site of visceral afferents from the 9th and 10th cranial nerves, including input from the arterial chemoreceptors, baroreceptors, stretch receptors in the heart and lungs, and many others. The location of the DRG within this tract suggests that the DRG may function to integrate afferent information for the control of breathing.
- Two types of inspiratory neurons in the DRG: type Ia are inhibited by lung inflation and Type Ib are excited by lung inflation. These appear to be related to the Hering-Breuer inflation and deflation reflexes, respectively.
- Ventral respiratory groups (VRG) - associated with nucleus ambiguus, para-ambiguus, nucleus retromabigualis - both inspiratory and expiratory cells. Function of VRG neurons is to project to distant sites and drive either spinal respiratory motorneurons (primarily intercostal and abdominal) or the auxiliary muscles of respiration innervated by the vagi, including structures of the larynx and pharynx. A few fibers from Bötzinger complex project to DRG.
- Respiratory rhythm generation - mechanism is still unknown,
but cells in pre-B
tzinger complex may act as pacemakers.
- Apneustic Center
- Location: In reticular formation of pons above the medullary respiratory center.
- If the brain is sectioned immediately above this level and the vagus nerves are transected get prolonged inspiratory efforts interrupted by occasional expiratory efforts (apneusis).
- Apneusis only occurs if the vagus nerves are also transected. This suggests that afferent information, from the vagus nerves (and possibly the spinal cord) may be important in preventing apneusis.
- Apneusis is probably a general phenomenon involving sustained discharge of medullary inspiratory neurons.
- The apneustic center may be the site of the normal "inspiratory cutoff switch." It is the site of projection of the various inputs that can terminate inspiration. Apneusis arises from inactivation of this inspiratory cutoff mechanism.
- Thus the apneustic center must stimulate inspiratory neurons and directly or indirectly inhibit expiratory neurons.
- Pontine Respiratory Groups (Pneumotaxic Center)
- Located in the upper pons (nucleus parabrachialis medialis; Kölliker-Fuse nucleus)
- If the brain is transected immediately above the pneumotaxic center, the pattern of respiration shows an essentially normal balance between inspiration and expiration.
- Electrical stimulation of the above structures results in synchronization of phrenic activity with the stimulus and premature switching of respiratory phases. Pulmonary inflation afferent information inhibits pneumotaxic center respiratory activity.
- The pneumotaxic center probably functions to "fine tune" the respiratory pattern, whether by setting the timing of the inspiratory cut off or by modulating the respiratory system's response to stimuli such as hypercapnia, hypoxia, and lung inflation. In any case, respiratory rhythmicity can exist without the pneumotaxic center.
- The pneumotaxic center then inhibits inspiration either by direct effects on the inspiratory neurons, or more likely by inhibiting the apneustic center.
- Spinal Pathways:
- Projecting axons from the DRG, VRG, cortex, and other supraspinal sites descend in the spinal white matter to influence phrenic, intercostal, and abdominal motorneurons of respiration
- At the level of the spinal respiratory motorneurons, there is integration of descending influences as well as the presence of local spinal reflexes that can affect these motorneurons. Ascending pathways in the cord can also influence respiration.
- Inspiratory and expiratory components appear to be separated in the cord.
- Descending axons with inspiratory activity excite external intercostal motorneurons and also inhibit internal intercostal motorneurons by exciting spinal inhibitory interneurons.
- Reflex mechanisms of respiratory control (See Levitzky pages 196-197 for complete list)
- Respiratory reflexes from pulmonary stretch receptors. (Hering-Breuer reflexes)
- Hering-Breuer inflation reflex:
- Stimulus - pulmonary inflation (a "stretch reflex")
- Receptor site - large and small airways (within smooth muscle)
- Afferent pathway - vagus
- Effect - apnea; bronchodilatation; (increased H.R., decreased SVR)
- Show very little adaptation
- Controversial whether the pulmonary stretch reflex is important in determining rate and depth of ventilation in adult man, since the central threshold is high (VT's > lL) during eupnea.
- May be important in babies.
- Hering -Breuer deflation reflex:
- Stimulus - pulmonary deflation
- Receptor site - lungs (probably also in airways)
- Afferent pathway - vagus
- Effect - hyperpnea
- Probably not too important under normal circumstances
- Paradoxical reflex of Head (partial block of vagus)
- Stimulus - pulmonary inflation
- Receptor site - lungs
- Afferent pathway - vagus
- Effect - inspiration
- First breath of newborn; sigh
- Other pulmonary reflexes affecting respiration:
- Mechanical or chemical irritation of airways (and alveoli?) Cause cough or hyperpnea and bronchoconstriction.
- Pulmonary embolism causes apnea or rapid shallow breathing; pulmonary vascular congestion causes hyperpnea. Receptors in pulmonary vessels (Type J receptors)
- Diving reflex (see Lecture on Diving Physiology).
- Cardiovascular - respiratory reflexes
- Arterial chemoreceptors
- Stimulus - low PaO2, high PaCO2, low pHa
- Receptor - carotid bodies, aortic bodies
- Afferent pathways - glossopharyngeal (carotid body) and vagus
- Effect - Hyperpnea, bronchoconstriction
- Arterial Baroreceptors (minor influence on respiration)
- Stimulus - high arterial blood pressure
- Receptors - carotid sinus, aortic arch
- Afferent pathway - glossopharyngeal, vagus
- Effect - Apnea
- Pharyngeal dilator reflex
- Stimulus - negative pressure in upper airway
- Receptor sites unknown - in nose, mouth, upper airway
- Afferent pathway - trigeminal, laryngeal, glossopharyngeal
- Effect - contraction of pharyngeal dilator muscles
- Important in preventing obstructive sleep apnea
- Muscle and tendon influences on respiration
- Receptors in diaphragm, intercostal muscles and tendons and joints of limbs can stimulate respiration.
- Pain - somatic pain causes hyperpnea:
- The vagus nerve and breathing
- Afferents
- Hering - Breuer and paradoxical reflex
- Sensory innervation of airways
- Aortic chemoreceptors and baroeceptors
- Efferents - bronchomotor, bronchial secretion (below recurrent laryngeals). Glottis (above recurrent laryngeals).
- Respiration after vagal section- slower and deeper due to loss of stretch reflexes. Main danger due to difficulty in swallowing and loss of cough reflex.
- Influences of higher center on breathing
- Maximum voluntary ventilation - highest
E attainable
- Speech, singing, etc.
- Emotional states
- Chemical control of breathing - a negative feedback system
- Arterial PO2, PCO2 and pH
- CSF pH and PCO2
- CO2
- Regulation of PaCO2 the most important input in the control of ventilation. (Remember, however, that CO2 changes will produce pH changes.)
- Powerful stimulus - The greatest respiratory stimulation that can be produced in nonexercising individuals is that produced by increasing PICO2. Much greater than lowering PIO2.
- Sensitive control - PaCO2 changes little (< lmmHg),
if at all, during exercise even though
ECO2 may be 10 times normal.
- Response to inspired CO2
- Increased PICO2
Increased
E
- Note steepest slope is between 5-10% CO2 in inspired
air (PaCO2
38-70 mmHg). Levels off above 10%.
- Above approximately 7% CO2 in inspired air effects become quite pronounced: vigorous respiratory stimulation, dyspnea, faintness, severe headache, and dulling of consciousness.
- Above about 15% CO2 inspired unconsciousness, muscular rigidity, tremors.
- With 20-30% CO2 inspired generalized convulsions.
- Although CO2 is normally a respiratory stimulant, acute very high PaCO2's (> 60-70 mmHg) produce respiratory depression.
- The response to lower inspired CO2 concentrations:
- Concentrations of CO2 producing PACO2's
of 38-50 (
PaCO2's in same range) linearly increase
E. Young man:
E = 2-5L/min/mmHg PaCO2; decreases with age: about ½ at 70 years of age.
- Note effect of PaO2: curve steeper and shifted to the left.
- Response may be decreased by chronic airway obstruction, sleep, and especially anesthesia, barbiturates, opiates.
- Metabolic acidosis shifts the curve to the left.
- Increasing
E increases removal of metabolically produced CO2.
PaCO2
Therefore, under normal circumstances: increased PACO2Increased
E
decreased PaCO2.
- Mechanism of CO2 influence on control of respiration:
- Both the peripheral chemoreceptors (carotid and aortic bodies) and central chemosensitive sites are stimulated by CO2.
- CO2 effects at each chemosensitive site may be due to either a (local) rise in PCO2 or H+ concentration.
- Central effects of PACO2 are much greater than the peripheral chemoreceptor effects. Probably about 80% is central, 20% is from arterial chemoreceptors. The breath to breath response originates from the arterial chemoreceptors.
- Arterial chemoreceptors:
- At normal levels of arterial PCO2, pH and PO2 there is considerable chemoreceptor impulse traffic over the carotid sinus nerve. All have similar response patterns to a given stimulus. No recruitment.
- In single fiber recordings each fiber appears to transmit the activity generated by decreased pHa, increased PaCO2 and decreased PaO2.
- Response nearly linear over PCO2 range of 20-60 mmHg
- Also stimulated by decreased blood pressure and by increased sympathetic activity, which presumably acts by causing a vasoconstriction, thereby reducing blood flow to the chemoreceptor.
- Central sites of CO2-induced stimulation:
- Central chemosensitive sites appear to be on the brain side of the blood-brain barrier. Response takes about 60 sec.
- CO2 can easily diffuse through the blood - brain barrier, but H+ ions and HCO-3 ions do not pass through easily. However, any change in PCO2 in the brain will lead to a proportional change in the H+ concentration. Thus the chemosensitive cells may actually be responding to H+ concentration changes.
- Under normally circumstances the brain is producing CO2. Brain CO2 level will be higher than that of arterial blood.
- In certain states (e.g. metabolic acidosis or alkalosis) blood H+ concentration and central H+ concentration may change in opposite directions. This is because of the extreme slowness with which fixed acids and bases cross the blood-brain barrier and the great ease with which CO2 traverses it. There may be active transport of HCO-3 across the blood brain barrier but it is slow.
- Hydrogen Ions
- Role of changes in H+ concentration on regulation of respiration are difficult to separate from CO2 effects.
- Response to H+ nearly linear from 20-60 nanequivalents per liter.
- O2.
- Response to alveolar hypoxia
- Little effect on respiration until PAO2 < 50 - 60 mmHg.
- Interaction with PACO2; response is enhanced when hypoxia is simultaneous with hypercapnia.
- The peripheral chemoreceptors appear to be entirely responsible for the ventilatory response to hypoxia.
- In the absence of the carotid chemoreceptors the effect of increasing degrees of hypoxia is a progressive depression of the central mechanisms of respiratory control, leading to complete respiratory failure.
- It appears that the aortic arch chemoreceptors alone are not able to sustain the increased ventilation in hypoxia.
- When the peripheral chemoreceptors are intact, the depressant effects of hypoxia on respiration can by overcome by the influences of nerve impulses reaching the respiratory centers from the chemoreceptors.
- The degree of the respiratory response to hypoxia is related to the change in O2 tension (PaO2) rather than the O2 content of arterial blood.
- Hypoxia alone (as in altitude) increases
E which leads to decreased PaCO2 and subsequent respiratory alkalosis.
- Mechanism of chemoreception by the carotid body unknown
- Certain enzyme poisons that block the formation of ATP stimulate the carotid body - Hypoxia may act in a similar manner.
- Carotid body has a very high blood flow (2000 ml/100 grams of tissue/min) and very small A-V O2 difference (0.15 ml O2/100 ml blood), even though is has one of the highest metabolic rates in the body.
- Two types of cytochrome a3 in the carotid body: One with a high affinity for O2 (which may maintain normal metabolism) and one with a low affinity for O2 (which may be associated with impulse generation).
- Relation between structure and generation of impulses.
- Glomus cells with nerve fiber in close proximity. Form reciprocal synapses so glomus cells are both pre- and post-synaptic to the sensory afferent nerves.
- Glomus cells high in dopamine, acetylcholine
- Ventilatory response to exercise:
- Immediate response - "neural component"
- Central Command
- Learned or conditioned reflex
- Direct connections from motor cortex-collaterals from motor neurons to muscles.
- Coordination in hypothalamus
- Proprioceptors or mechanoreceptors in limbs (probably not muscle spindles or Golgi tendon organs.)
- Subsequent Increase
- During moderate exercise
- Arterial chemoreceptor
- PO2 - usually no change in mean
- PCO2 - usually no change in mean
- [H+] - usually no change unless cross anaerobic threshold
- [K+] - minor role
- Oscillations in arterial PO2 and PCO2 probably important
- Metaboreceptors in exercising muscles
- Nociceptors - H+, K+, bradykinin, arachidonic acid
- Cardiac receptors
- Venous chemoreceptor - venous PO2
, pH
, PCO2
- Temperature receptors -
temperature stimulates breathing
- During exercise severe enough to exceed anaerobic threshold (lactic acid buffered by HCO-3 produces CO2)
- Arterial chemoreceptors
- PCO2
- [H+]
- Central chemoreceptors PCO2 H+
- Potentiation of chemoreceptor responses during exercise.
Last updated Tuesday, November 25, 2003 2:47 PM
LSUHSC is an equal opportunity educator and employer.
The statements found on this page are for informational purposes only. While every effort is made to ensure that this information is up-to-date and accurate, for official information please consult a printed University publication.
The views and opinions expressed in this page are strictly those of the page author. The contents of this page are not reviewed or approved by LSUHSC.
This page is maintained by webmaster-arc: mgiaim@lsuhsc.edu